Meteodyn will participate at the ACP Resource & Technology Conference to be held if Phoenix, Arizona, from September 30th to October 2nd, with two posters presentations about Meso-Microscale Coupling for Wind Resource Modelling and about a new technology for Offshore Wind Resource Assessment.
Meteodyn is expanding its presence in North America, led by Minh-Thang DO, Technical Manager and Business Development Engineer of Meteodyn North America.
Minh-Thang will be representing Meteodyn at the upcoming ACP Resource & Technology Conference 2024, where he will deliver two poster presentations.
Don’t miss the opportunity to explore their abstracts below and connect with him in person to get your questions answered.
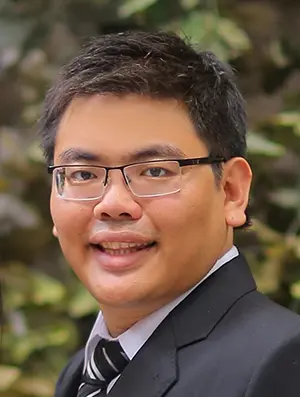
2 poster presentations
Wind Resource Modelling in Complex Terrain through Meso-micro Coupling Method in Considering Thermal Stability Effects
Coastal regions offer an ideal environment for wind farms due to the consistent and strong winds that blow in from the sea. Coastal wind farms can achieve high energy yield because the sea breeze tends to be more consistent and stronger than inland winds, resulting in increased efficiency and a more reliable power supply. The expansive open spaces and proximity to populated areas make these locations attractive for the development of wind energy projects, leading to a growing trend in the construction of wind farms along coastal areas.
In studying wind flow in coastal regions, attention should increasingly focus on the impacts of both microscale topography and regional climate conditions, the thermal stability effect. These effects are coupled between them and can be correctly reproduced only with an appropriately designed model chain covering both microscale and mesoscale phenomena. In Meteodyn WT, the mesoscale-microscale coupling method combines mesoscale models which capture the climate conditions and introduce them in the micro-scale model, with microscale model that focus on the terrain complexity to improve the wind resource modelling at the coastal area. The statistic wind profiles from meso-scale model are used not only as the initial and boundary conditions but also as forces in the computational domain to maintain the statistic meso-scale wind profiles in meso-scale zone. The Monin-Obukhov length obtained from the mesoscale data is used to choose thermal stability classes.
Here, we investigate a coastal site equipped with several meteorological masts using various methodologies: the Computational Fluid Dynamics (CFD) modelling approach, the mesoscale-microscale coupling approach, and the multi mesoscale modelling approach. The results indicate that the mesoscale simulation tends to underestimate the wind in this coastal region. Both Coupling method and Multi meso method demonstrate improvements in horizontal extrapolation between masts.
Notably, in the absence of met mast measurements, the wind speeds obtained through the microscale approach are more sensitive to the reference provided by the mesoscale simulation compared to the mesoscale-microscale coupling approach. Therefore, incorporating mesoscale data in the CFD modelling using the mesoscale-microscale coupling approach can help reduce errors, compared to relying on a single reference point.
While various approaches, such as multi meso modeling, microscale modeling, and meso-micro coupling modeling, each demonstrate their advantages in wind modeling, the choice depends on factors such as climate conditions, the topography conditions and measurement availability.
Increasing the accuracy of offshore wind resource assessment with high-resolution satellite imagery
Offshore wind resource assessment is a challenge due to scarcity of measurements at hub height. It is usually estimated based on a combination of mesoscale modeling and offshore metocean observations, such as offshore met masts and fixed or floating lidars. Lidars generally provide short-term measurements at a single location. Numerical models can provide long time series and cover a large area, but they have a strong dependency on the numerical parameterization of the atmospheric boundary layer and tend to flatten heterogeneities and extremes because of the filtering and dampening of numerical schemes. These approaches can be combined with statistical Measure-Correlate-Predict (MCP) techniques to take advantage of both (IEC 61400-1 2019). But an important level of uncertainty about the actual offshore wind resource remains, particularly in coastal areas where the environmental conditions are complex. Offshore blowing winds, short-fetch sea conditions, gravity waves, etc., induced by orography effects from the coast and thermal impacts in the land-sea atmospheric transition result in strong air-sea and land-sea interactions. These are known to be challenging conditions to model, even at high resolution with a mesoscale model.
On the other hand, spaceborne synthetic-aperture radar (SAR) provides observations of the Earth surface in almost all-weather conditions (except intense precipitation), night and day, regardless of the cloud cover. Over the ocean, it enables visualizing fine spatial details of the complex atmospheric flows, especially in coastal regions. SAR measures the surface backscattered signal from the sea surface roughness, i.e., the centimetre-scale waves directly related to surface wind stress. The corresponding surface wind speed is retrieved from geophysical model functions that are empirical relationships between the backscattered signal, angles of the radar signal, and wind direction and wind speed. A 20-year database of European SAR provides worldwide surface wind measurements at a 500-m resolution over 250-km-wide areas: they provide accurate statistics and atlas despite the low revisit rate of the satellite (i.e., a few days over an area). Here, surface wind speeds from the European Sentinel-1 missions are used: they are daily quality-controlled, calibrated and validated by the SAR Mission Performance Centre Service through the Copernicus Program implemented by the European Space Agency.
SAR observations have recently been used to derive surface wind atlas (e.g., in the US, in Europe and in Cyprus) by capturing complex air-sea and land-sea interactions in off- and near-shore locations. To address altitudes relevant to offshore wind projects, a vertical extrapolation is needed. De Montera et al. (2022) developed an advanced methodology, called SARWind, to provide a comprehensive site characterization of a dedicated offshore wind project. It relies on the synergy between high-resolution satellite observations, in-situ data, and mesoscale modelling. The methodology is based on three steps: (i) derivation of the 10-min SAR surface winds from SAR sea surface roughness, and a site-independent machine learning algorithm based on the US NDBC network (43 metocean buoys) to correct SAR winds due to biases inherent to the characteristics of the satellite sensors and wind retrieval methodology, (ii) extrapolation up to a few hundred meters based on a second machine learning algorithm trained with in-situ observations and physical parameters from a high-resolution mesoscale model related to e.g. atmospheric stability, and (iii) a final post-processing step to correct for low temporal sampling of the SAR database (one passage every two days for one satellite) and retrieve wind statistics (e.g. mean wind speed, Weibull parameters). The resulting output is a 500-m resolution wind atlas in a large (>3000 km²) offshore and/or coastal area where strong coastal gradients will be accounted for, or it can integrate directly the estimation of the extractible wind power.
The extrapolation algorithm has been trained on datasets from 12 lidars in the North Sea and validated over 28 lidars in Germany, the Netherlands, Belgium, France, US (East and West coasts), China. For instance, when comparing to short-term lidars measurements from 40 m to 200 m in South Brittany, France, the bias between extrapolated SAR wind speed and lidar measurements is very low (less than 0.1 m/s).
These unique coverage, precision and resolution from SAR measurements bring great benefits such as wind atlases with spatial heterogeneities for the characterization of wind conditions in coastal/offshore regions, hence helping in early screening of development zones and designing lidar campaigns. While the aforementioned extrapolation methodology does not require any on-site measurements, we illustrate here the possibility to use on-site Lidar data to improve the accuracy of the SAR-based wind resource, therefore taking advantage of the accuracy of Lidar measurements and of the spatial distribution assessed by SAR. A new methodology to provide temporal and spatial MCP IEC-compliant products is under development.